Selected applications of UTOPIA-NMR.
​

(a) Simplified pulse scheme for the unified acquisition of a 1H-detected TROSY-HNCA (parent) and a 13C-detected CON (child) experiment. (b,c) Superposition of spectra acquired conventionally (black) with data acquired in the UTOPIA setup (red). (b) Extract of the 3D HNCA, (c) 2D CON. Note that splittings in the CON spectrum due to CO-Ca J-couplings have been removed using maximum entropy deconvolution, resulting in well-resolved signals for nearly all residues in Bcl-xL (including all prolines). (d) Application of UTOPIA-NMR to large biological systems. 13C-detected FLOPSY spectrum recorded on OmpX in nanodiscs (200 mM perdeuterated OmpX at 700 MHz). Only the child experiment is shown (red) and compared to a conventional spectrum (black). Labels show easily identifiable Ca-Cb correlations in the transmembrane region of OmpX (red residues in model above). (e,f) Typical NMR analytics spectra recorded on a sample of 2 mM unlabeled sucrose (2D 1H,1H TOCSY (e) and 1D 13C (f)). In all cases the unified acquisition of parent and child allowed data acquisition in exactly half of the time as compared to the respective conventional setups.
UTOPIA setup for small molecules
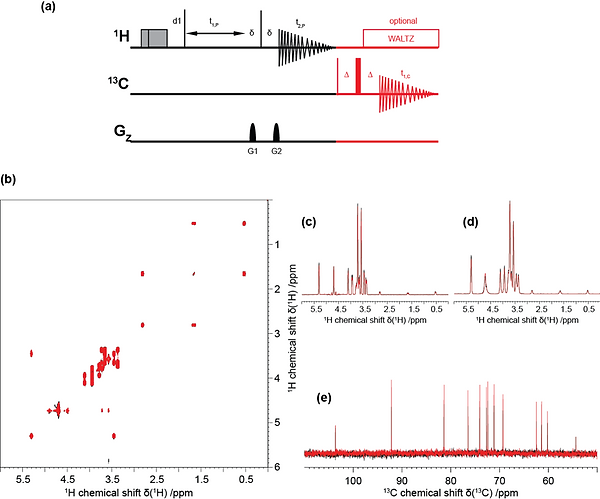
UTOPIA setup for small molecules recorded on 2 mM unlabelled sucrose in 90% H2O and 10% D2O.
(a) Pulse sequence scheme for the unified acquisition of a 1H,1H-COSY spectrum (black) and a 90°-13C spectrum (red).
Both parent and child pulse sequences were used with the standard parameters (i.e. pulses, phases, delays, etc.) as found in the Bruker library. Note that the refocusing step (with a delay Δ = 20 μs) in the 13C-detected experiment was introduced due to RF ringdown effects, which only appeared in one of three spectrometers on which we tested the sequence. In general, it may hence be favorable to omit refocusing.
Note that, in the shown setup the UTOPIA child has slightly higher signal intensity as compared to the conventional spectrum due to the effects of the parent experiment. Despite both the UTOPIA child and the respective reference spectrum were acquired without NOE enhancement (i.e. in the absence of power gated decoupling), the presence of the UTOPIA parent will lead (as expected) to increased intensity of the 13C-detected experiment. This enhancement will, however, be lower (for protonated carbons) than the one obtained with a standard NOE-enhanced experiment. Nevertheless, in this setup the UTOPIA parent is not disturbed by the child, meaning that the child (although slightly lower in intensity as a conventional NOE enhance experiment) is obtained for free. Alternatively, a power gated decoupling period could be included in the UTOPIA sequence (directly after 1H detection). The duration of this period will however prolong the experimental time and therefore effectively lead to a reduction in the parent sensitivity (signal per time). In this respect it also provides a possibility to adjust the relative sensitivity of parent and child to some extend.
​
(b) Superposition of the conventional (black) and interleaved (red) 2D 1H,1H-COSY spectra. (c, d) Superposition of the corresponding projections in the direct and indirect dimension, respectively. (e) Superposition of the conventional (black) and interleaved (red) 1D 13C spectra.
Note that the 1H decoupling during 13C detection will interrupt 1H relaxation and hence, if short recycle periods are used, may lead to a decrease in the 1H detected signal intensity in comparison with a conventional experiment. This will be in particular true in cases were 13C-T2 values are in the order of 1H-T1 values and relaxation delays are specifically optimized for the 1H-T1 properties of the sample. However, in routine screening of small molecules inter-scan relaxation delays are often not specifically optimized for each sample, instead fixed values of e.g. 1-4 s are used. This was also done for the data shown here, where a relaxation delay of 2 s was set for the 13C detected experiment. Our data show that in this view and for the sucrose sample used, the 1H detected experiment can be recorded for free during the acquisition of the 13C detected experiment, resulting in identical information content in exactly half of the measurement time as compared to the conventional data.

(a) Pulse sequence scheme for the unified acquisition of a 1H,1H-TOCSY (black) and a 90°-13C (red). Both parent and child pulse sequences were used with the standard parameters as found in the Bruker library. As in the example above, a refocusing setup was added in the 13C-detected experiment to tackle RF ringdown effects. (b) Superposition of the conventional (black) and interleaved (red) 2D 1H,1H-TOCSY spectra. (c, d) Superposition of the corresponding direct and indirect projections, respectively. (e) Superposition of the conventional (black) and interleaved (red) 1D 13C spectra. Data were recorded on 2 mM unlabeled sucrose. (Also see comment regarding refocusing and T1 relaxation above).
UTOPIA setup for complex systems

(a) Pulse sequence scheme for the unified acquisition of a 1H,15N-NOESY-TROSY (black) and a 13C,13C-FLOPSY (red). (b+c) shows extracts of the corresponding parent experiment recorded in a conventional (b) and in an UTOPIA-NMR setup (c). No significant differences are observed regarding the appearance and intensity of NOESY peaks in both spectra. Both parent and child pulse sequences were used with the standard parameters as found in the Bruker library. Note that in this study no scheme was introduced to eliminate 1JCC in the FLOPSY spectra, instead acquisition and processing parameters were selected that resulted in line widths above 50 Hz, i.e. not resolving 1JCC. Nevertheless, if desired, techniques to reduce/eliminate 1JCC could be incorporated into the UTOPIA setup. Experimental conditions were 350 μM Bcl-xL, 25°C, Bruker Avance III HD at 600 MHz

Pulse sequence scheme for the unified acquisition of a 1H,15N-HNCA (black) and a 13C,15N-CON (red). Both parent and child pulse sequences were used with the standard parameters as found in the Bruker library. Note that the relaxation delay (d1) is placed just before the child experiment which renders the usage of the standard (composite pulse) 1H decoupling scheme in the child inefficient, as it would lead to significant loss of signal in the parent experiment. Instead, two 1H π-pulses (one decoupling pulse in the middle of the 15N evolution period and one recovery pulse directly after) were used and the time between the pulses was kept as short as possible to minimize 1H magnetization losses. Using this sequence we do not observe reduced sensitivity for the parent or reduced resolution in the child.
​
​
For more information:
​